Research areas:
Shock waves, characterized by discontinuity in temperature, pressure, and density, are ubiquitous in numerous engineering fields. Shock waves can arise due to explosions in different mediums (e.g., air, water), projectile impacts in materials (such as bullet impact), movement of hypersonic vessels (such as ICBM- Intercontinental Ballistic Missiles, atmospheric reentry of spacecraft), meteorite impacts, etc. In these extreme loading situations, the medium plays a significant role in the propagation and reflection of shock waves passing through it. In certain situations (depending upon shock intensity), the medium also undergoes changes (evidenced by phase transitions and chemical reactions). Some snippets of our group’s multiyear study exploring this field are presented below, along with references to relevant publications (for a detailed exposure to the topic).
Underwater shock propagation and reflection:
Underwater mines do not need to explode in contact with the surface of ships/submarines. If they explode at a certain distance, they create shock waves in addition to cavitation bubbles, which can result in “hull whipping” and eventual breakdown of the entire frigate/corvette/destroyer. Even though there exist studies for underwater shock wave propagation for far-field applications, there were no studies (till the time we did our study) on near-field explosions in which the intensity of the shock can be quite intense. Within the context of continuum mechanics, considering water to be a non-linear compressible medium (modeled using the Mie-Gruniessen Equation of State), we developed novel theories for impulse transmission to a rigid plate, demonstrating the beneficial effects of fluid-structure interaction. Our theoretical and numerical results (vonNeumann-Richtmyer algorithm) have been asymptotically validated against existing theoretical results in the acoustic range (which models far-field explosion-induced shock waves) for better design of shock mitigation structures.
Most of these theories for shock propagation typically consider normal incidence, but in reality, there can be oblique incidences. Considering different wedge angles for appendages and Mach no., a comprehensive study (using Shock polar diagrams) identifies regions of no-shock reflection, regular and irregular reflection regimes, sonic incident criteria, and detachment criteria.
Phase transition in Water:
As a shock wave propagates through water, it changes the molecular structure of water, and interestingly, liquid water crystallizes into ice VII-like form (density = 1.65 g/cc @ 2.5 GPa and 250C). Prior Russian and American literature (primarily experimental) demonstrates this phase transition. It needs to be noted that ice VII, even though not prevalent on Earth, this form of water is expected to form cores of the icy planetary giants (Uranus, Neptune) and plays a vital role in the development of gravitational forces in those planets (similar to that of the iron core for Earth). The question we were after for this study is how does a disordered structure (liquid water) become ordered (crystal ice VII-like form) for this material in which the main governing forces are hydrogen bonds between the molecules. Based on our diffusion studies at the molecular scale (reduced translational diffusion similar to ice VII for eight coordinated lattice structures but high rotational diffusion resulting in a random arrangement of hydrogen atoms), we were able to show that for shocked water, the resultant crystal lattice structure even though is similar to ice VII but the arrangement of hydrogen atoms in the water molecule are arbitrary. Thereby, it can be regarded as a “plastic crystal phase” at the junction between liquid water and ice VII in the phase diagram of water. Apart from the scientific value of the study, it needs to be noted that for shock mitigation design studies, this physical change (associated with phase transition) will result in changes to the equation of state of the medium, thereby having implications with regards to the impulse transmission to the structure.
Shock induced Chemistry in Air:
The topic of shock propagation and reflection in air has been well-studied within the aerospace community (Refer to classic books by Ben-Dor). However, one topic has been elusive. The coefficient of reflection (CR – ratio of reflected pressure to the incident shock pressure) has been observed to approach 20 in 1940 studies during the Manhatten project. The acoustic approximation for shock waves in the air provided a value of CR equal to 2, which has been the basis for shock mitigation design standards. An MIT group, considering the non-linear compressibility of air, was able to show the value of CR to reach 8. However, there was still a lack of theoretical understanding of how CR can be more than 8. Within the continuum mechanics framework and based on consideration of real gas equation of state for Air (van der Waals approximation) in which all vibrational, electronic degrees of freedom (dof) were considered for air molecules in addition to translational and rotational dof (typically considered for ideal gas EoS considered in prior studies), we were able to demonstrate that the Cr value can reach 16. Bounds in peak overpressure were demonstrated where vibrational and electronic contributions to the dof become important. Still, one theoretical limitation was that it only considered non-interacting gaseous particles. In reality, these gaseous molecules will undergo chemical reactions resulting in new chemical species and the breakdown of existing species (demonstrated through molecular studies of shock-induced chemical reactions in air).
Funding Acknowledgements:
US: Office of Naval Research Global, Office of Naval Research
India: Naval Research Board, Indian Space Research Organization
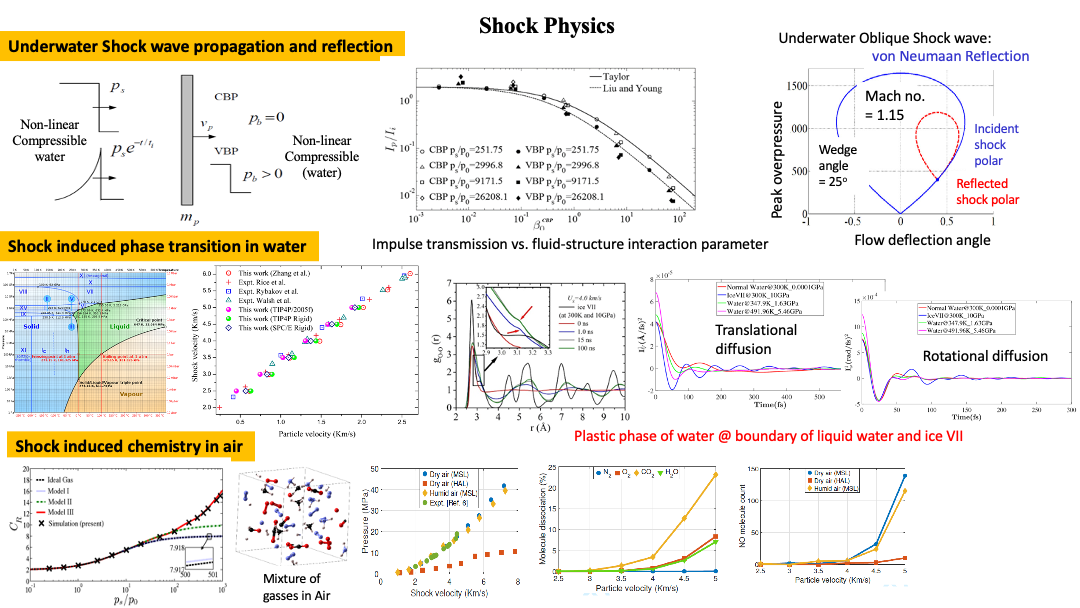
The energy input to a material must be dissipated based on energy conservation. This dissipation in energy typically results in the formation of defects within materials. These defects in materials could be point defects (vacancies and interstitials), line defects (dislocations), planar defects (grain boundaries), and volume defects (stacking fault tetrahedra, precipitates). Phase transformations and chemical reactions can also occur in materials if the energy input to the material is significantly high within a short time period (as in a shock or radiation exposure). These defect formation and evolution eventually describe the mechanistic principles of deformation in materials resulting in demonstrations such as plasticity in metals. Our group has spent significant time and effort trying to understand these defect formations and evolution in different solid materials – metals (FCC, BCC, and HCP), polymers, ceramics, and even minerals. Observing the numerous mechanisms governing defect formation and evolution in different materials is fascinating and vary widely. For example, while dislocations govern responses in metals, the uncoiling of molecular chains governs responses in polymers.
Defects in Metals:
Primarily metals exhibit simplistic periodic crystal lattices such as FCC, BCC and HCP. Deformation mechanism for 3 different types of metals (based on their lattice structure) have been elucidated in the study: Cu for FCC, Nb for BCC and Ti for HCP. Depending upon the intensity of energy input (based on magnitude and time duration) in the material, numerous sessile dislocation loops could be observed in Cu such as evidenced by Hirth-locks, Lomer-Cotrell locks, Cross-Slips and Stacking fault tetrahedra. As the intensity of the shock wave input into the material is increased, FCC to BCT phase transitions are observed. Our theoretical predictions of phase transformations (based on MD and DFT studies) for single crystal Cu have been eventually validated by experimental researchers for polycrystalline Cu materials. For HCP type materials in which twins are well known to occur, we have demonstrated formation of different variants of tension and compression twins under different types of loading conditions. Non-Schmid behavior was also observed in numerous twin variants. BCC materials are typically the most complex out of the three since they exhibit numerous slip planes (in contrast to FCC and HCP) and are also known to violate Schmid law. Shock studies of Nb revealed the competing dislocation and twin activities in these materials. In addition to that, formation of new phases were also demonstrated. Typically, any shock studies in metallic materials are associated with spallation behavior which has also been shown in the recent study on Nb.
Defects in Non-metals:
Crystal structure of Non-metals are typically more complex than conventional metals. One of our studies explored Si which exhibits a cubic diamond crystal structure at ambient temperature and pressure conditions. As the crystal is hydrostatically compressed, it undergoes changes to different complex lattice forms (as has been mentioned in experiments). We carried out a MD study to develop necessary equations for identification and characterization of those complex crystal phases for single crystal Si subjected to hydrostatic loading and exhibiting different phase transitions with increase in load intensity.
Defects in Ceramics:
The overall crystal structure of Ceramics might be simplistic such as those demonstrated for metals but in these situations we have more than one element. Moreover, in addition to metallic bonding, some amount of covalent bonding is observed in most ceramics which eventually results in directionality of properties. We have investigated one such Ceramic, which is used in many different fields ranging from semiconductors to ballistic protection – SiC in which the orientational anisotropy in response was demonstrated for the material. Efforts have also been made in the MD based study to show dislocation “like” activities within the material subjected to different types of loading situations by tracking individual atoms within the material.
Defects in Minerals:
Within the periodic crystal lattice structures, minerals exhibit some fairly complex structures, as are evident in numerous minerals that we have studied. Some of these minerals, also have water in their interlayers or interspaces which complicates the structure-property relations. The presence of these intermolecular water was observed to play a significant role in mechanical response of these minerals as they are subjected to different types of loading conditions.
Defects in Polymers:
Contrary to the periodic structures exhibited by crystal lattices, polymers exhibit an amorphous chain like molecular structure. Application of loading, results in coiling and/or uncoiling of these chain-like structures, which are eventually manifested as plateau type regions in the stress-strain response of the material. Polymers are also highly susceptible to temperature and thereby their response depends significantly upon the temperature at loading (above or below the glass transition temperature). Our study in polymers is not only restricted to simplistic coiled structures exhibited by thermoplastic polymers but also includes thermoset which are typically formed upon crosslinking reactions between two polymers eventually forming a chain network. Under conditions of extreme loading, polymers also undergo chain scission depending upon the individual binding energies of different bonds such as C-C, C-H bonds and others. Lack of transport properties (thermal and electrical conductivity) is also typical for that of polymers in which there is absence of free electrons as we observe for metals.
Funding Acknowledgements:
US: Office of Naval Research, Army Research Office, Air Force Office of Scientific Research
India: Naval Research Board, Indian Space Research Organization, Ministry of Human Resources and Development, Department of Science and Technology
Chemistry involves changes in electronic environment of materials resulting in formation and scission of bonds. These chemical events may be initiated by numerous physical stressor such as shock, radiation, electromagnetic exposure along with placing two chemical compounds near to each other (such as observed in corrosion, oxidation, hydration etc.). Our studies involves chemical changes in materials as they are subjected to different types of stressors. The studies are not only limited to synthetic materials but also includes biological living materials. We typically are interested in chemical reaction kinetics and have observed numerous examples of catalytic events. So far our studies have involved primarily adsorption and decomposition. Some examples of our studies include:
Cement Hydration:
Cement (typically obtained as a powder) reacts with water to form a man-made rock “concrete” which is widely used in infrastructures. Even though this widely known process is well understood with regards to the initial and final product of the reaction, questions still remain about the intricacies of the chemical reactions which can help us to control the reaction kinetics. In our studies at the level of quantum chemistry, we eventually found out the different chemical mechanisms influencing these chemical reactions in which calcium silicate crystals (alite, belite) undergoes hydration to convert to hydrated calcium silicates. In the process there occurs an oligomerization of the silicate chains which accounts for the hardness observed in “concrete” or “cementitious material”.
Swelling Clay:
Red Lateritic soil is found in many parts of the globe, especially in the areas between the tropics. An interesting characteristic of these soils is that they absorb water to “swell” (typically observed during rainy seasons) and also release water to “deswell” (typically observed in hot seasons). This interesting phenomenon (similar in characteristic to that of swelling/deswelling of hydrogels) is brought about by an intricate competition between hydrogen bond networks as well as metal-ligand interactions. The role of different metal cations influencing this characteristic has been explored in one of our quantum-chemistry based investigations.
Decomposition of energetic materials:
Energetic materials find use in explosives as well as rocket propellants. Conventional organic energetic materials such as nitramines have complex molecular structures. The potential energy surface of decomposition for caged nitramines (such as CL20) has been investigated in the quantum-chemistry study which can give us an estimation of the amount of energy released and the stepwise progression of decomposition.
Crosslinking and catalysis in epoxy-amine reactions:
One class of adhesives are epoxy-amines in which during curing the epoxide ring breaks in presence of amine eventually resulting in crosslinking. This well-known chemical reaction pathway has been studied to look for catalysts in the process. In addition to water, certain metal cations in small quantities were found to catalyze the reactions. The quantum-study delves into the details of the chemical reaction pathway for these systems.
Adsorption of resins on material surfaces:
Adsorption of a material (gas or liquid) on a material surface requires an in-depth understanding of the electronic environment of the specific material (adsorbent) surface in which the chemical reactivity of the global surface as well as the local elements within that surface needs to be assessed prior to initiating the chemical reaction between the two materials. The purpose of the quantum study was to investigate the chemical pathways (potential energy surface) of resin adsorbing on alumina surface (dry and hydrated) as well as silica surfaces.
Excited state reaction pathways for biomolecules:
Biomolecules (such as proteins, amino acids, nucleobases for DAN/RNA) undergo conformational changes as they are subjected to radiation. The conformational changes are associated with excited state pathways. The excited state pathway involving conical intersections were investigated for nucleobase pairs. The study has applications to origins of life, exposure of biomolecules to UV and different radiation environments as in nuclear reactors as well as in space (such as cosmic radiations).
Chemical reactivity changes under irradiation:
Gamma radiation typically results in formation of radicals thereby changing the molecular structure of minerals. The change in molecular structure is associated with change in chemical reactivity.
Funding Acknowledgements:
US: Office of Naval Research, Army Research Office, Air Force Office of Scientific Research
India: Ministry of Human Resources and Development
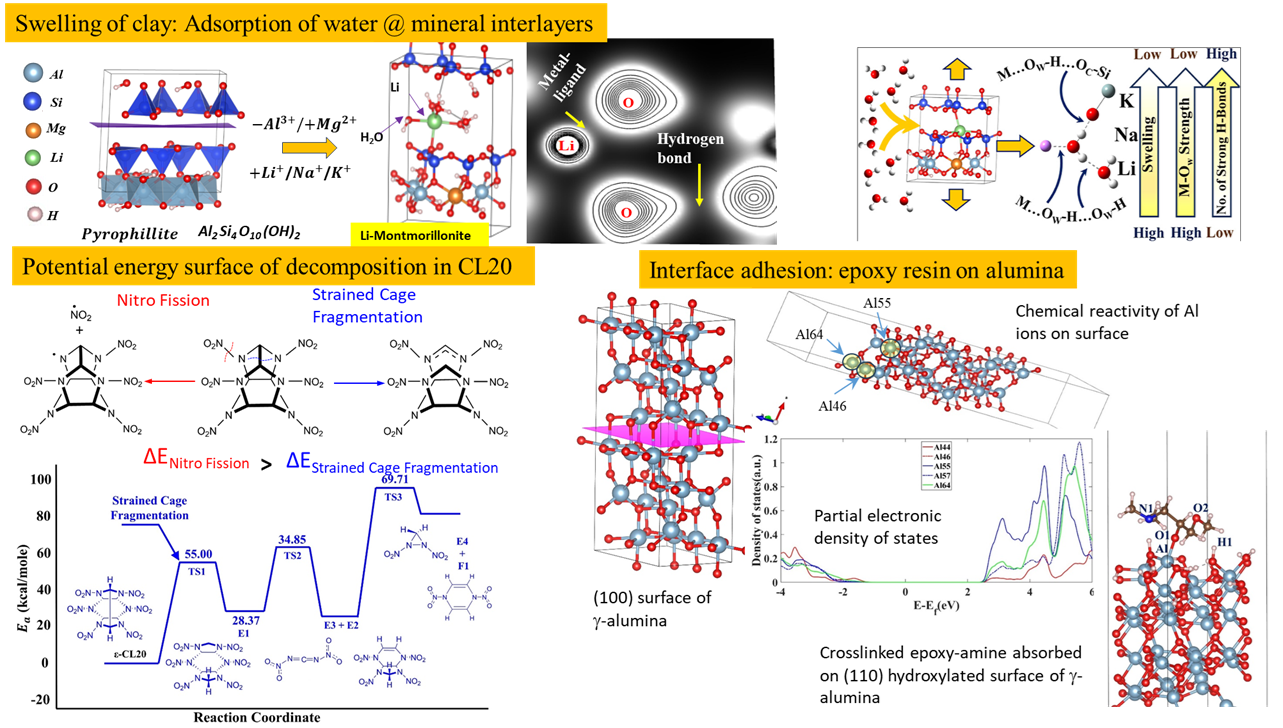
Investigation of materials may not be always required at the level of molecules or atoms. At a larger length scale, these materials contain large amounts of defects (since its difficult to obtain/produce pristine materials with no defects). Many a times, the behavior of the materials influencing a global response needs to be addressed at the level of continuum. Numerous studies (theoretical and experimental) that has been performed by our group includes the following:
Impact on materials:
Projectile impact on materials results in formation of craters in the target as well as ejecta formation. These impacts also result in development of shock waves through the target material. If the target material is thin compared to the kinetic energy being imparted by the projectile, it can also result in full penetration. Impact studies are not only important from a defense perspective in which governing mechanisms needs to be fundamentally understood for design of armors against bullets (MEDE program) but are also required for planetary studies such as the DART mission by NASA and numerous other space agencies around the world. Our group has performed numerical studies to develop and understand the mechanisms behind bullet penetration as well as kinetic impactor utilized in the DART mission.
Delamination of sandwich composites:
Sandwich composites comprise of foams and fiber composites (glass, carbon, Kevlar etc.). These are used for design of hull structures of ships to even aerospace and space structures. The ability of foams to damp out vibrations as well as absorb blast loads are necessary for design of these advanced lightweight composites for numerous applications ranging from transportation sector to even packaging. Even though these types of composites are widely utilized, one major problem associated with these materials is the possibility of delamination between the two dissimilar materials under an applied load — terminology coined in literature as “delamination”. Our group has performed extensive studies to fundamentally understand the mechanisms of delamination, identify them using numerous fiber-optic based sensor design and also mitigate them using numerous techniques such as shear-keys and nano-doping.
Fatigue and Fracture in cementitious composites:
Cementitious composites are the binding materials in modern day infrastructure. Our research revealed that proper addition of fiber reinforcements in traditional cementitious composites as well as alternative green (based on waste products from other industries instead of cement such as fly-ash, blast furnace slag etc.) cementitious composites can result in significant improvements to the mechanical response of these materials in addition to durability. A fiber-reinforced cementitious composite compressive strength can easily reach and even exceed the strength of concrete material (which are composed of coarse aggregates in addition to cementitious composites).
Seismic response of reinforced concrete beam-column joints:
Apart from anthropogenic disasters, many of disasters are natural such as earthquakes. As part of my PhD study, I was involved in development of models for seismic response of reinforced concrete beam column joints. The study of natural disasters (especially earthquakes) was carried out by PEER. Structural mechanics-based models for joints along with their constitutive relationships combining numerous mechanisms was developed as part of the study.